4.1 Technical Principles and Status Quo
This chapter discusses the applications of hydrogen, focusing on its energetic and material uses. Material use involves utilizing hydrogen's chemical properties for reactions with other elements, while energetic use converts hydrogen's energy into electricity and heat. The production of e-fuels is mentioned briefly. Hydrogen is mainly used as a material in the chemical industry and crude oil refining. Future applications in industries like steelmaking and plastics production are also explored.
Energetic use
In today's energy system, the energetic use of hydrogen plays a subordinate role. In the course of increased sector coupling, however, especially green hydrogen could also be increasingly used as an greenhousegas-neutral energy source in the future, for example in the mobility sector. The energetic use of hydrogen can basically take place in two different processes. In addition to classical combustion, there is the possibility of using hydrogen in fuel cells. In the following, the basic principles of these two technologies for the energetic use of hydrogen are described.
Fuel Cells
In a fuel cell, the chemical energy bound in the hydrogen is converted directly into electrical energy. Due to the direct conversion without a thermal process - as it takes place in a classic gas turbine, for example - high efficiencies can be achieved with the help of fuel cell technology. Depending on the design, fuel cells can be operated not only with hydrogen but also with a hydrogen-containing fuel gas, such as natural gas (CH4). The design of a fuel cell is similar to that of an electrolytic cell and is shown schematically in the illustration below.
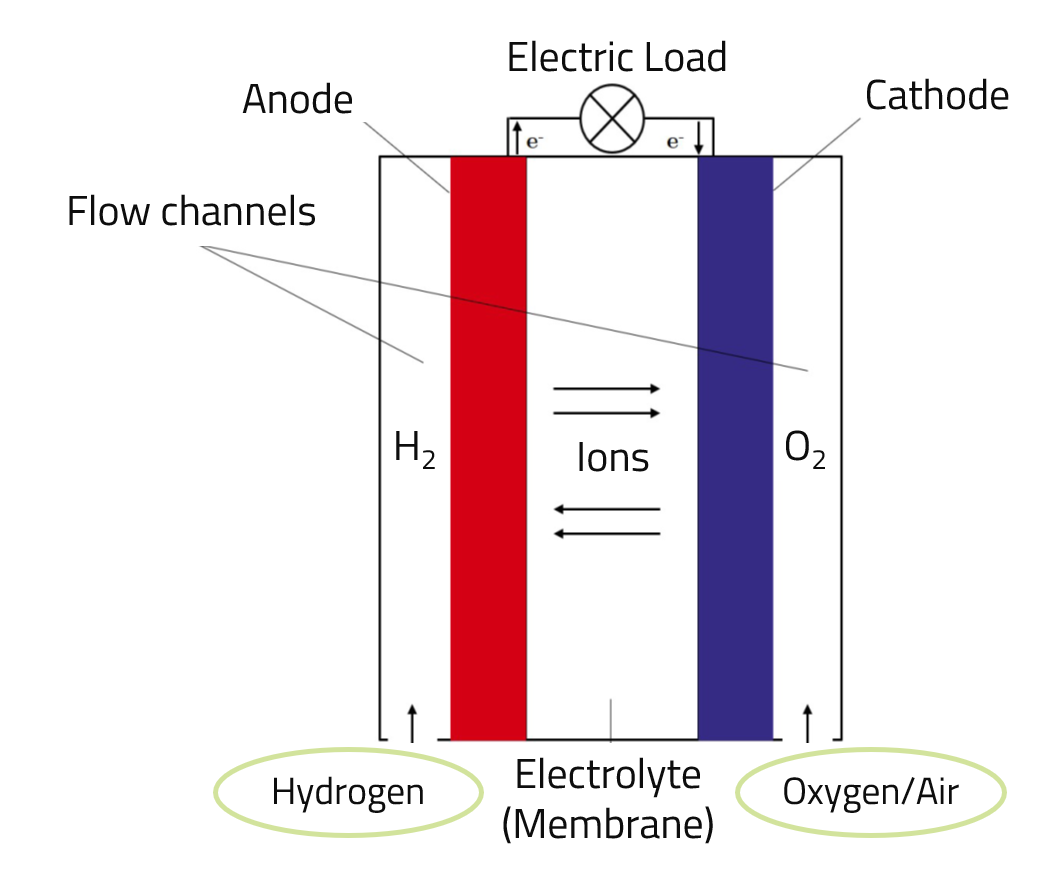
H2 --> (2 H+) + (2 e-)
These electrons are taken up by the anode and travel via an electrical conductor to the cathode. The flow of electrons can be used as an electric current via an external circuit.
2 H2 + O2 --> 2 H2O + Electricity + Heat
In practice, fuel cells achieve electrical efficiencies of up to 60 % and are thus significantly more efficient than conventional thermal combustion processes. In addition to electrical energy, the waste heat generated during the reactions can also be used. Similar to electrolysis cells, in practice a large number of individual fuel cells are interconnected to form so-called stacks in order to generate higher voltages.
Combustion
Hydrogen combustion is with regard to the underlying reaction principles comparable to the combustion of natural gas. However, hydrogen combustion has some special characteristics:
- No CO2 is produced during the combustion of hydrogen. The only reaction product of the combustion is water vapour.
- The temperature that results from the combustion of hydrogen is higher than that of natural gas combustion.
- Due to the higher combustion temperatures, the formation of nitrogen oxide (NOx) is enhanced and, without countermeasures, is higher than with the combustion of natural gas. Nitrogen oxides are pollutants and have a negative effect on people's lung function.
- When hydrogen is burnt, the spread of the flame into areas not intended for this purpose can lead to problems. The reason for this spread is the very high flame velocity of hydrogen (several times higher compared to natural gas), which results in a more difficult to control combustion of the gas. The flame velocity describes the speed at which unburnt gas spreads in the flame.
- Compared to natural gas, hydrogen combustion is connected to some additional safety risks. For example, less energy is required for the ignition of hydrogen, which means that spontaneous combustion can occur more easily if hydrogen accidentally emerges (e.g. in the case of damaged pipelines). In addition, the flame that is formed during the combustion of hydrogen is almost invisible. This leads, for example, to the fact that fire brigades depend on infrared cameras to identify and extinguish the source of the fire in accidents where hydrogen is burning.
The challenges for safe hydrogen combustion can be solved by technological adaptations. Thus, the development and optimisation of combustion systems for hydrogen is currently being worked on. Some manufacturers already offer smaller gas turbines and gas engines that are suitable for operation with hydrogen.